Projects

AMPA receptor is visualized by tagging it with GFP.
Protein Trafficking and Long-term Potentiation
When hippocampal neurons fire intensely, the synaptic transmission between the neurons is strengthened for a long-term. This phenomenon is called long-term potentiation (LTP) and is considered as a cellular counterpart of learning and memory. But how synaptic transmission is enhanced for long-term had not been clarified.
We tested a novel hypothesis that LTP induction recruits new AMPA type glutamate receptor molecules to the synapse, thereby increasing the postsynaptic sensitivity to released glutamate. Using both optically and electrophysiologically tagged glutamate receptors, we showed that LTP induction delivers new AMPA receptor molecules to the synapse [1] [2] [3]. Ca2+/calmodulin-dependent protein kinase II (CaMKII) and a putative PDZ domain binding protein are both required for this process. The trafficking is not limited to AMPA receptor. We found multiple proteins are trafficked to the synapse in temporally ordered fashion[4].
Instead, it does not require phosphorylation of AMPA receptor itself[2]. As opposed to widely prevailing view, the proportion of phosphorylated AMPA receptor is too low to explain synaptic plasticity[5]. Therefore, what triggers AMPA receptor trafficking and what retains the trafficked receptor are still questions to be answered.
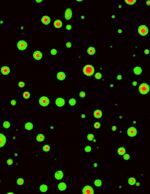
Activation of CaMKII induces segregation of NMDA receptors (green) and AMPA receptor (red).
Liquid-liquid phase separation and synaptic plasticity
Ca2+/calodulin-dependent protein kinase II (CaMKII) is a serine/threonine protein kinase critically involved in synaptic plasticity in the brain[7]. It is highly concentrated in the postsynaptic density (PSD) fraction, exceeding the amount of any other signal transduction molecule, almost comparable with cytoskeletal proteins[7]. Because kinase signaling can be amplified by catalytic reaction, it does not have to exist in such large quantity. Also CaMKII forms unique dodecameric structure not seen in other kinases. These remained mysteries.
We recently found that Tiam1, an activator of small G-protein Rac, forms persistent complex with CaMKII[8]. Not only that, we found that CaMKII interacts with multiple other proteins and cross-link them together (unpublished). From these results, we speculated that CaMKII may undergo liquid-liquid phase separation, a phenomena where protein solution is segregated into condensed and diluted phase, like water and oil[9]. CaMKII did not undergo separation in inactive form. However, when activated with Ca2+, CaMKII undergoes phase-separation and forms a condensed phase. Furthermore, it triggers the condensation of other proteins as well[6]. This property of CaMKII may be a mechanism to trigger translocation and accumulation upon induction of LTP[10].
Molecular Mechanisms of Structural Plasticity of Dendritic Spines
Synaptic activity also induces postsynaptic structural enlargement of dendritic spines, tiny protrusions on dendrite where synapses are formed [11][12][4](Movie 1). By using a FRET sensor, we found that actin is polymerized rapidly in response to a LTP-inducing stimulation protocol moved the equilibrium towards polymerization [13] [14]. The change persists notably for at least 30 minutes after the stimulation. The regulation of actin can provide a mechanism for synaptic, activity-dependent delivery of postsynaptic proteins as well as cytoskeletal role.
Among molecules implicated in synaptic plasticity, we found biochemical evidence that CaMKII is capable of bundling F-actin through a stoichiometric interaction[15]. Activation of CaMKII detaches it from F-actin, thereby allowing modification of F-actin during LTP[16]. CaMKII serves as a central molecule with both signaling and structural role in the excitatory synapse[17][7].
Structural LTP[4]
When red dot appears, glutamate was uncaged locally on the tip of dendritic spine of a neuron expression GFP.Actin polymerization and expansion of dendritic spine[13]
Gating of sLTP by CaMKII[16]
Autophosphorylation of CaMKII dissociate it from F-actin, thereby allowing its modification.
Dynamic Behavior of Cellular Memory Engram
In hippocampus, there are neurons which fires when an animal is at specific position, called place cells. They are considered to encode memory of the animal’s position. How they are formed and how long they last have not been fully elucidate. In order to address these questions, we set up a virtual reality system where an animal can run through while we observe the hippocampal neuronal activity by using two-photon Ca2+ imaging [18] [19] [20] . The Ca2+-event are automatically detected from images and deduced into spike patterns[21]. Using this approach, we are trying to elucidate the dynamic behavior of cellular memory engram.
Mouse virtual reality system[19]
References
- ↑ 1.0 1.1
Shi, S.H., Hayashi, Y., Petralia, R.S., Zaman, S.H., Wenthold, R.J., Svoboda, K., & Malinow, R. (1999).
Rapid spine delivery and redistribution of AMPA receptors after synaptic NMDA receptor activation. Science (New York, N.Y.), 284(5421), 1811-6. [PubMed:10364548] [WorldCat] [DOI] [Google Scholar] - ↑ 2.0 2.1
Hayashi, Y., Shi, S.H., Esteban, J.A., Piccini, A., Poncer, J.C., & Malinow, R. (2000).
Driving AMPA receptors into synapses by LTP and CaMKII: requirement for GluR1 and PDZ domain interaction. Science (New York, N.Y.), 287(5461), 2262-7. [PubMed:10731148] [WorldCat] [DOI] [Google Scholar] - ↑
Shi, S., Hayashi, Y., Esteban, J.A., & Malinow, R. (2001).
Subunit-specific rules governing AMPA receptor trafficking to synapses in hippocampal pyramidal neurons. Cell, 105(3), 331-43. [PubMed:11348590] [WorldCat] [DOI] [Google Scholar] - ↑ 4.0 4.1 4.2
Bosch, M., Castro, J., Saneyoshi, T., Matsuno, H., Sur, M., & Hayashi, Y. (2014).
Structural and molecular remodeling of dendritic spine substructures during long-term potentiation. Neuron, 82(2), 444-59. [PubMed:24742465] [PMC] [WorldCat] [DOI] [Google Scholar] - ↑
Hosokawa, T., Mitsushima, D., Kaneko, R., & Hayashi, Y. (2015).
Stoichiometry and phosphoisotypes of hippocampal AMPA-type glutamate receptor phosphorylation. Neuron, 85(1), 60-67. [PubMed:25533481] [PMC] [WorldCat] [DOI] [Google Scholar] - ↑ 6.0 6.1
Hosokawa, T., Liu, P.W., Cai, Q., Ferreira, J.S., Levet, F., Butler, C., Sibarita, J.B., Choquet, D., Groc, L., Hosy, E., Zhang, M., & Hayashi, Y. (2021).
CaMKII activation persistently segregates postsynaptic proteins via liquid phase separation. Nature neuroscience, 24(6), 777-785. [PubMed:33927400] [WorldCat] [DOI] [Google Scholar] - ↑ 7.0 7.1 7.2
Kim, K., Saneyoshi, T., Hosokawa, T., Okamoto, K., & Hayashi, Y. (2016).
Interplay of enzymatic and structural functions of CaMKII in long-term potentiation. Journal of neurochemistry, 139(6), 959-972. [PubMed:27207106] [WorldCat] [DOI] [Google Scholar] - ↑
Saneyoshi, T., Matsuno, H., Suzuki, A., Murakoshi, H., Hedrick, N.G., Agnello, E., O'Connell, R., Stratton, M.M., Yasuda, R., & Hayashi, Y. (2019).
Reciprocal Activation within a Kinase-Effector Complex Underlying Persistence of Structural LTP. Neuron, 102(6), 1199-1210.e6. [PubMed:31078368] [PMC] [WorldCat] [DOI] [Google Scholar] - ↑
Hayashi, Y., Ford, L.K., Fioriti, L., McGurk, L., & Zhang, M. (2021).
Liquid-Liquid Phase Separation in Physiology and Pathophysiology of the Nervous System. The Journal of neuroscience : the official journal of the Society for Neuroscience, 41(5), 834-844. [PubMed:33472825] [PMC] [WorldCat] [DOI] [Google Scholar] - ↑
Liu, P.W., Hosokawa, T., & Hayashi, Y. (2021).
Regulation of synaptic nanodomain by liquid-liquid phase separation: A novel mechanism of synaptic plasticity. Current opinion in neurobiology, 69, 84-92. [PubMed:33752045] [WorldCat] [DOI] [Google Scholar] - ↑
Hayashi, Y., & Majewska, A.K. (2005).
Dendritic spine geometry: functional implication and regulation. Neuron, 46(4), 529-32. [PubMed:15944122] [WorldCat] [DOI] [Google Scholar] - ↑
Bosch, M., & Hayashi, Y. (2012).
Structural plasticity of dendritic spines. Current opinion in neurobiology, 22(3), 383-8. [PubMed:21963169] [PMC] [WorldCat] [DOI] [Google Scholar] - ↑ 13.0 13.1
Okamoto, K., Nagai, T., Miyawaki, A., & Hayashi, Y. (2004).
Rapid and persistent modulation of actin dynamics regulates postsynaptic reorganization underlying bidirectional plasticity. Nature neuroscience, 7(10), 1104-12. [PubMed:15361876] [WorldCat] [DOI] [Google Scholar] - ↑
Okamoto, K., & Hayashi, Y. (2006).
Visualization of F-actin and G-actin equilibrium using fluorescence resonance energy transfer (FRET) in cultured cells and neurons in slices. Nature protocols, 1(2), 911-9. [PubMed:17406324] [WorldCat] [DOI] [Google Scholar] - ↑
Okamoto, K., Narayanan, R., Lee, S.H., Murata, K., & Hayashi, Y. (2007).
The role of CaMKII as an F-actin-bundling protein crucial for maintenance of dendritic spine structure. Proceedings of the National Academy of Sciences of the United States of America, 104(15), 6418-23. [PubMed:17404223] [PMC] [WorldCat] [DOI] [Google Scholar] - ↑ 16.0 16.1
Kim, K., Lakhanpal, G., Lu, H.E., Khan, M., Suzuki, A., Hayashi, M.K., Narayanan, R., Luyben, T.T., Matsuda, T., Nagai, T., Blanpied, T.A., Hayashi, Y., & Okamoto, K. (2015).
A Temporary Gating of Actin Remodeling during Synaptic Plasticity Consists of the Interplay between the Kinase and Structural Functions of CaMKII. Neuron, 87(4), 813-26. [PubMed:26291163] [PMC] [WorldCat] [DOI] [Google Scholar] - ↑
Okamoto, K., Bosch, M., & Hayashi, Y. (2009).
The roles of CaMKII and F-actin in the structural plasticity of dendritic spines: a potential molecular identity of a synaptic tag? Physiology (Bethesda, Md.), 24, 357-66. [PubMed:19996366] [WorldCat] [DOI] [Google Scholar] - ↑
Sato, M., Kawano, M., Ohkura, M., Gengyo-Ando, K., Nakai, J., & Hayashi, Y. (2015).
Generation and Imaging of Transgenic Mice that Express G-CaMP7 under a Tetracycline Response Element. PloS one, 10(5), e0125354. [PubMed:25946002] [PMC] [WorldCat] [DOI] [Google Scholar] - ↑ 19.0 19.1
Sato, M., Kawano, M., Mizuta, K., Islam, T., Lee, M.G., & Hayashi, Y. (2017).
Hippocampus-Dependent Goal Localization by Head-Fixed Mice in Virtual Reality. eNeuro, 4(3). [PubMed:28484738] [PMC] [WorldCat] [DOI] [Google Scholar] - ↑
Sato, M., Mizuta, K., Islam, T., Kawano, M., Sekine, Y., Takekawa, T., Gomez-Dominguez, D., Schmidt, A., Wolf, F., Kim, K., Yamakawa, H., Ohkura, M., Lee, M.G., Fukai, T., Nakai, J., & Hayashi, Y. (2020).
Distinct Mechanisms of Over-Representation of Landmarks and Rewards in the Hippocampus. Cell reports, 32(1), 107864. [PubMed:32640229] [PMC] [WorldCat] [DOI] [Google Scholar] - ↑ Takekawa, T., Asai, H., Ohkawa, N., Nomoto, M., Okubo-Suzuki, R., Ghandour, K., Sato, M., Hayashi, Y., Inokuchi, K., Fukai, T.
Automatic sorting system for large calcium imaging data
bioRxiv, 2017 [DOI][Google Scholar]